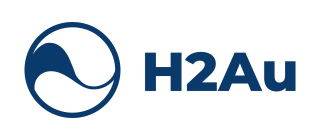
H2Au is committed to the exploration and extraction of natural hydrogen. We leverage geological and geophysical expertise and methods developed for the oil and gas and mining industries to identify and develop natural hydrogen. Our multidisciplinary team includes experts from the petroleum, mining, and radioactive waste management industries, providing a unique blend of skills to tackle the challenges of hydrogen exploration (hard rock + soft rock).
The Science
Natural hydrogen can be generated through several geological processes, such as radiolysis, the breakdown of water by natural radioactivity (Warr et al., 2019). However, H2Au focuses primarily on serpentinization due to its apparently rapid kinetics and significant hydrogen generation potential (under ideal conditions), coupled with the ability to focus exploration around a defined ultramafic protolith. Serpentinization occurs when ultramafic rocks, react with water, transforming olivine into serpentine, magnetite, brucite and generating hydrogen as a byproduct. This reaction is highly efficient, particularly under elevated temperatures ranging from 200 to 320°C, thus serving as a significant source of natural hydrogen (McCollom et al., 2016). However, hydrogen generation can also occur at lower temperatures, especially in environments where olivine is predominantly composed of the fayalite end member (McCollom et al., 2022) – see Figure 1. The main conditions for effective serpentinization include having the right protolith, an adequate water supply, and optimal pressure and temperature conditions (Klein et al., 2013).
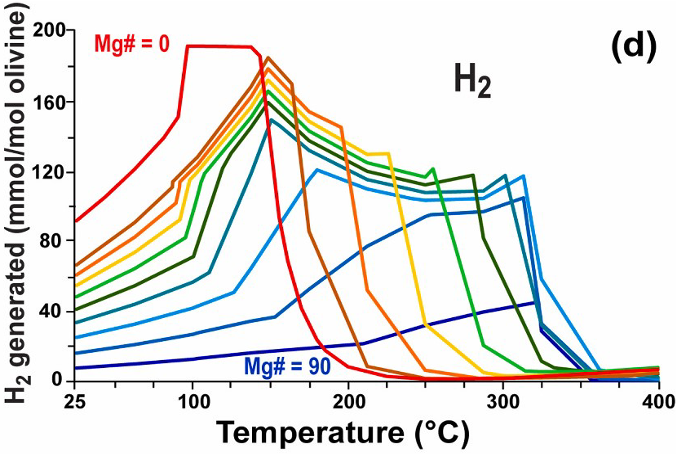
Figure 1 – H2 generation prediction for serpentinization of olivine with a range of Fe / Mg contents as a function of temperature. After McCollom et al., 2022
One effective way to generate hydrogen is by the serpentinization of ultramafic rocks within Precambrian greenstone belts. These ancient formations are rich in olivine and provide an ideal setting for serpentinization when reached by water through faults and fractures. They are also readily accessible for study at outcrop across many ancient cratonic terranes such as the Kaapvaal Craton in South Africa (Anhaeusser 2006) – Figure 2. The interaction between meteoric water and olivine-rich rocks such as these greenstones has the potential to produce significant amounts of hydrogen (Hutchinson et al., 2024). The key to this model is identifying ultramafic intrusions with the right thermal history and suitable geological conduits to allow water access, creating an efficient system for hydrogen generation and migration.
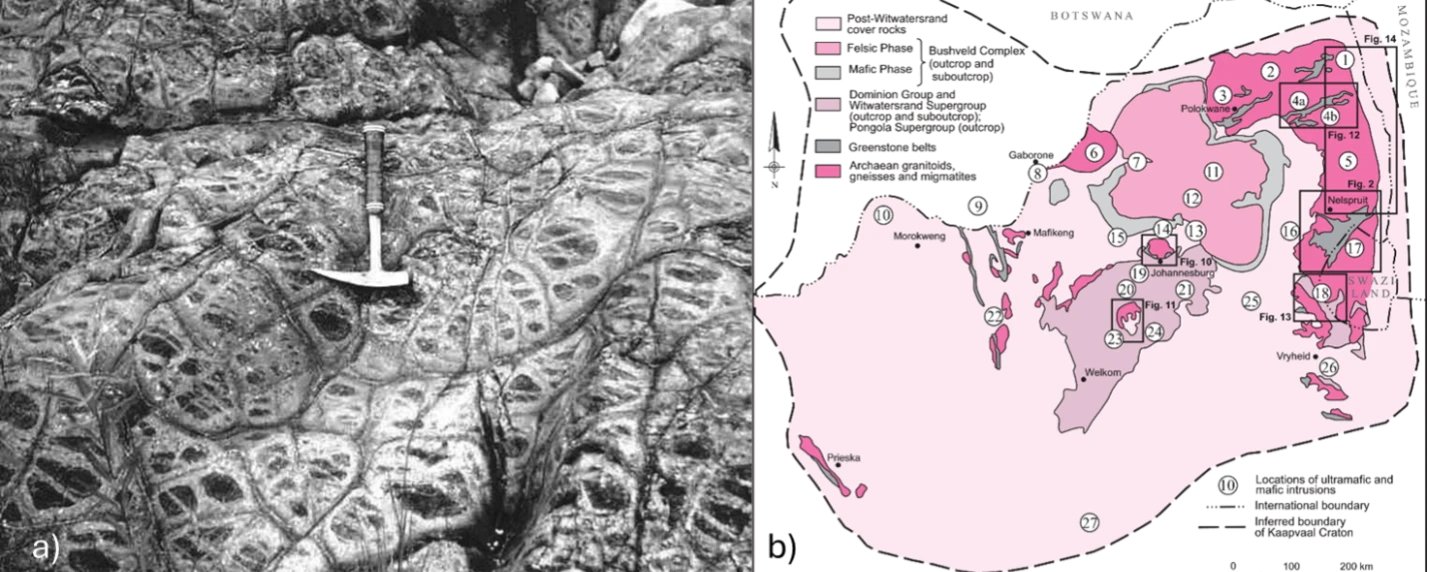
Figure 2 – a) Remnants of Serpentinised Dunite from the Barberton greenstone intrusion, South Africa and b) Locations of ultramafic and mafic intrusions of the Kaapvaal Craton, South Africa. Anheausser 2006
Regional Screening
Our exploration process begins with a comprehensive screening study to identify areas with high potential for hydrogen production. This involves analysing regional scale geological and geophysical data to co-locate the key hydrogen system elements into a play fairway. The main steps in this process include protolith identification, where we delineate the ultramafic protolith including extrapolating from outcrop beneath sedimentary cover and assess its potential for hydrogen generation (thermal and structural history). We also analyse water sources, identifying major faults and aquifers that can provide the necessary water supply for the serpentinization reaction. Geophysical data, such as gravity and magnetic surveys, are crucial in mapping the composition and structure of the basement rocks. Higher density ultramafic rocks have a strongly positive gravitational signature, however their serpentinization leads to a strongly positive magnetic response and a reduction in the density (gravity signature)(Falcon-Suarez et al., 2017, Prieto 1998). Additionally, well log data and core samples are analysed to determine geochemistry of the protolith, its remaining potential and any presence of hydrogen within the overlying section.
Hydrogen migration and entrapment are critical aspects of the screening study. Once hydrogen is generated, it must migrate via advection to a suitable reservoir rock where it can be trapped and stored – this could be either an overlying or adjacent sedimentary package or fracture systems within the surrounding basement. This process involves identifying major faults and fractures that facilitate hydrogen movement. Suitable reservoir rocks are those with sufficient porosity to accumulate hydrogen, while sealing rocks are impermeable layers that trap hydrogen, preventing it from escaping. The migration-retention system works similarly to hydrocarbon systems (Jackson et al., 2024), with hydrogen migrating through porous media and being trapped by capillary forces in low-permeability rocks. Despite its greater mobility, hydrogen can be sealed by similar lithologies that trap methane, allowing for significant column heights to build under suitable conditions (Hutchinson et al., 2024) Figure 3.
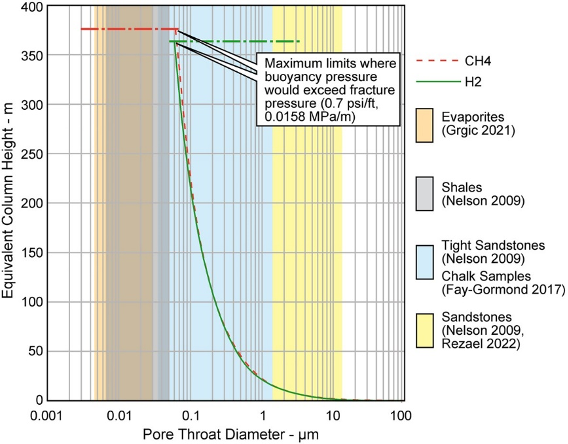
Figure 3 – Calculated attainable column heights for Methane and Hydrogen gas in relation to pore throat diameter of the overlying seal (based on capillary thresholds and buoyancy effects with a gas-water contact at 1000m). Note the close overlap between Methane and Hydrogen due to similar capillary entry pressure thresholds up to the fracture pressure thresholds for each gas. (Hutchinson et al., 2024)
Mapping Hydrogen Hotspots
The screening study is an extensive process that spans from the initial assessment of hydrogen generation potential to the final ranking of prospective sites using a common geological risking process, qualified by the ‘Italian Flag Method’ to distinguish high risk from high uncertainty. This method involves a detailed assessment of various factors that influence the potential success of a hydrogen system. These factors include the quality of the protolith and its thermal history, the availability of water for serpentinization and the presence and effectiveness of suitable reservoir rocks and sealing rocks. Each factor is evaluated according to the current knowledge of the system. If there are irrefutable indications in favour of the protolith, for example, this factor will be rated very highly, and the Italian flag will be more green. If there are still uncertainties, the rating will be lower, but the colour will be white. If there is information proving that this factor is very poor, the rating will be very low, and the colour will be red. This can result in prospects with a lot of white, indicating significant uncertainty, and if we decide to proceed in this direction, the pre-drill work program will be designed to address these uncertainties.
Conversely, a mainly green flag indicates a very good prospect to follow, while a mainly red flag should be ranked low. By evaluating these criteria, we can rank potential sites and focus our efforts on the most promising areas. This method allows us to prioritise exploration areas based on a comprehensive evaluation of geological and geophysical data, and can be applied to regions where hydrogen may not yet have been discovered (i.e. green field plays). The screening also provides a more rigorous model to support (or otherwise) any historical hydrogen occurrences indicated in well or mining data.
In addition to the geological screening, a detailed evaluation of licensing, offtake potential, access and environmental sensitivity are completed to ensure that the project can be commercially sustainable and valuable.
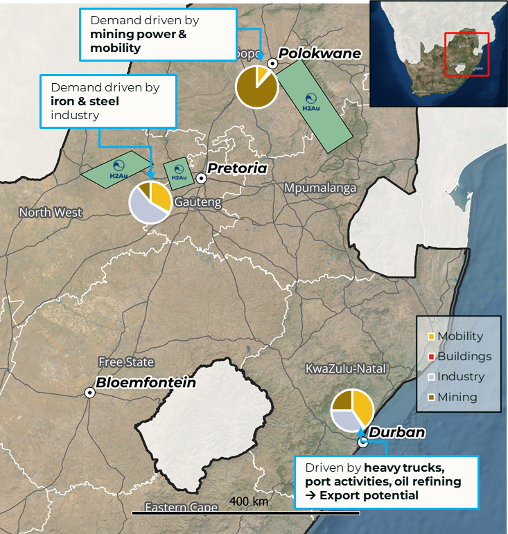
Figure 4 – H2Au Permit locations in South Africa (green). Pie charts are situated at proposed hydrogen hub locations where there is a high demand for decarbonisation and clean hydrogen.
This approach has been successfully applied by H2Au in various regions, most recently in South Africa, where we have pursued exciting plays associated with the Transvaal sequence (Figure 4). Our team collaborates with local authorities in charge of subsurface extraction, universities, and other subsurface stakeholders, including mining companies, to acquire valuable data and insights, ensuring that we leverage all available information and expertise to optimise our exploration efforts.
H2Au- Science led, Commercially driven.
References:
Anhaeusser, Carl. (2006). Ultramafic and mafic intrusions of the Kaapvaal craton. The Geology of South Africa. 95-134.
Falcon-Suarez, I., Bayrakci, G., Minshull, T. A., North, L. J., Best, A. I., Rouméjon, S., & IODP Expedition 357 Science Party. (2017). Elastic and electrical properties and permeability of serpentinites from Atlantis Massif, Mid-Atlantic Ridge1. Geophysical Journal International, 211(2), 686-699.
Jackson, O., Lawrence, S. R., Hutchinson, I. P., Stocks, A. E., Barnicoat, A. C., & Powney, M. (2024). Natural hydrogen: sources, systems and exploration plays. Geoenergy, 2(1), 2024-002
Klein, F., Bach, W. and McCollom, T.M. 2013. Compositional controls on hydrogen generation during serpentinization of ultramafic rocks. Lithos, 178, 55-69.
McCollom, T.M., Klein, F., Robbins, M., Moskowitz, B., Berquo´, T.S., Jöns, N., Bach, W., and Templeton, A. 2016. Temperature trends for reaction rates, hydrogen generation, and partitioning of iron during experimental serpentinization of olivine. Geochimica et Cosmochimica Acta, 181, 175-200.
McCollom, T.M., Klein, F., and Ramba, M. 2022. Hydrogen generation from serpentinization of iron-rich olivine on Mars, icy moons, and other planetary bodies. Icarus, 372, 114754.
Prieto, C. (1998). Gravity and magnetic signatures of various geologic models—An exercise in pattern recognition. Geologic Applications of Gravity and Magnetics: Case Histories. GeoScienceWorld Books.
Warr, O. Giunta, T., Ballentine, C., and Lollar, B.S., 2019. Mechanisms and rates of 4He, 40Ar and H2 production and accumulation in fracture fluids in Precambrian Shield environments. Chemical Geology,530, 119322.